PLANT LECTINS
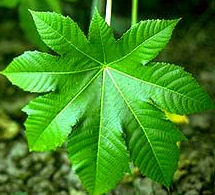
The high toxicity of castor beans was recognized during the last century when the extract was found to agglutinate a suspension of erythrocytes of different animal species. Since then, lectins were studied and extracted from plants, including fungus and lichens, as well as in animals.
Lectins are glycoproteins of 60,000-100,000 MW that are known for their
ability to agglutinate (clump) erythrocytes in vitro. There are over 400,000
estimated binding sites for kidney bean agglutinin on the surface of each
erythrocytes. Lectins are found in most types of beans, including soybeans.
Reduced growth, diarrhea, and interference with nutrient absorption are
caused by this class of toxicants. Different lectins have different levels
of toxicity, though not all lectins are toxic, though no all are toxins.
The bright scarlet seeds of precatory bean Abrus
precatorius contain the highly toxic glycoprotein, abrin. Less toxic
lectins can be fatal if ingested in high amounts. Some of such lectins
is concanavalin A from Concanavalia ensiformis (jack bean). Others
may exhibit no hemagglutinating activity as in the case of ricin from castor
bean and yet it is one of the most toxic substances.
The terms phytohemagglutinins, phytagglutinins, and lectins are used interchangeably. Lectins-containing plants have been found in many botanical groups including mono- and dicotyledons, molds and lichens, but most frequently they have been detected in Leguminoseae and Euphorbiaceae. They may exist in various tissues of the same plant and have different cellular localizations and molecular properties.
Lectins interaction with certain carbohydrate is very specific. This interaction is as specific as the enzyme-substrate, or antigen-antibody interactions. Lectins may bind with free sugar or with sugar residues of polysaccharides, glycoproteins, or glycolipids which can be free or bound (as in cell membranes). The term lectin refers to the specificity of the reaction (legere = to choose).
One of the major interest in this class of glycoproteins is the therapeutic use against HIV-1. Jacalin, a plant lectin, is found to completely block human immunodeficiency virus type 1 in vitro infection of lymphoid cells. This activity of the jacalin is attributed to its ability to specifically induce the proliferation of CD4+ T lymphocytes in human.
[Lectins in Food] [Functions of Lectins] [Lectins Structure] [Lectins in Plant Tissues] [Toxicity of Lectins] [Detoxification] [Lectins and Cancer] [References] [Return to list of toxicants]
Lectins in Foods
The ability to agglutinate human erythrocytes or representatives of human indigenous microflora was detected in 29 of 88 food items. Many foods contained substantial amounts of agglutinating activity, and lectins extracts could be diluted several folds and still produce agglutination. Great variation was observed in agglutination activity in the same food item purchased from different stores or from the same store on different days. Sometimes a food that possessed substantial activity on one day was found to have little or even no activity on other day.
A survey of the fresh and processed foods found lectins in about 30% of the food stuffs tested, including such common foods as salad, fruits, spices, dry cereals, and roasted nuts. However, dry heat may not completely destroy lectin activity. Hemagglutinating activity is found in the processed wheatgerm, peanuts, and dry cereals. Several lectins are resistant to proteolytic digestion e.g., wheatgerm agglutinin, tomato, lectin, and navy bean lectin.
Functions of Lectins
Not much is known about the functions of lectins in the organism they are formed. There is evidence that lectins may be involve in the recognition between cells or cells and various carbohydrate- containing molecules. This suggests that they may be involved in the regulating physiological functions. They seem to play an important role in the defence mechanisms of plants against the attack of microorganisms, pests, and insects. Fungal infection or wounding of the plant seems to increase lectins. In legumes, the role of lectins in the recognition of nitrogen-fixing bacteria Rhizobium genus, which have sugar-containing substances, has received a special attention.
Binding Nitrogen-Fixing Bacteria to legume Roots: among the possible functions of plant lectins is their participation in binding nitrogen-fixing bacteria to legume roots. In this reaction bacteria of the genus Rhizobium adhere to the surface of differentiated roots cells and are then internalized into the root hair to form nitrogen-fixing nodules. The symbiosis is specific in that certain species of Rhizobium can only associate with a particular species of legume. Such observations were made from the studies that were done on soybean Rhizobium japonicum and the clover Rhizobium trifolii. Other functions of lectins in plants may include:
- Enzymes (but unknown substrate)
- Storage of proteins
- Defense mechanism
- Cell wall extension
- Mitogenic stimulation
- Transport of carbohydrates
- Packaging and/or mobilization of storage materials
Lectins Structure
One major property of lectins is their specific saccharide- binding sites. Some lectins are composed of subunits with different binding sites. These include the lectin from the red kidney bean, Phaseolus vulgaris. It is composed of two different subunits combined into five different forms of noncovalently bound tetramers. Since subunits have very different specificities for cell surface receptors, each combination is considered to have a different function. The specificity of the binding sites of the lectins suggest that there are endogenous saccharide receptors in the tissues from which they are derived or on other cells or glycoconjugates with which the lectin is specialized to interact.
Metal Binding Sites
Biological activity of the lectins may be attributed to the metal ions which are the essential part of the native structure of most leguminous lectins. The most studies and fully sequenced lectin is concanavalin A. The metal binding sites of the concanavalin A are situated in the amino terminal part of the polypeptide chain. In this lectin, each subunit has aspartic 10 and 19, asparagine 14, histidine 24, serine 34, glutamic acid 8, and tyrosine 12 that are involved in the binding to one calcium and one magnesium ion.
Lectins of soybean, peas, faba bean, lentils, and sainfoin have amino acids that are involved in metal binding, which are conserved. The exception is of the tyrosine residue at position 12 of concanavalin A which is replaced with by phenylalanine in the other legume lectins.
Hydrophobic Sites
The stability of the native structure of most lectins is thought to be caused by the hydrophobic interactions. Such hydrophobic sites, forming cavities in the lectins structure, may play an important biological role. The hydrophobic binding sites of auxins, or cytokinin and adenine, for instance, by concanavalin A may enhance the functions of lectins on the plant life cycle.
Glycosylation Sites
Despite the generalization that most lectins are considered glycoproteins, concanavalin A, lentils lectin, and wheatgerm agglutinin contain no covalently attached carbohydrates. However, non-glycoprotein lectins are believed to be synthesized as glycosylated precursors. This is supported by the following observation:
- pro-concanavalin A is an inactive glycoprotein from which the glycosidic side chain is removed during post-translational processing.
- Non-glycoprotein wheatgerm agglutinin molecules are produced by removing a carboxyl terminal glycopeptide from the glycosylated precursor during post-translational processing.
All glycoprotein lectins contain a peptide sequence: asparagine- X-threonine/serine, which is characteristic of glycosylation sites. These sequences are different in the non-glycoprotein lectins. Also, peptide sequences, which in one glycoprotein lectin contain the glycosidic side-chains, are not necessarily conserved in another glycoprotein lectin. This may suggest that the biological activity of the lectins may not be determined by carbohydrate part of their structure.
Carbohydrate Binding Sites
Lectins differ markedly in their sugar-binding specificity. A sequence participating in carbohydrate-binding site of concanavalin A, for instance, are poorly conserved in other lectins.
The three dimensional structures of lectins can be used to show the structural similarities and homologies of legume lectins. When the secondary structure of Vicieae lectins, for instance, is compared with those concanavalin A, an identical or homologous B- turn structures are found in these lectins. Hydropathic profiles of two chains and single-chain lectins are superimposed, and concanavalin A has three domains that appear in some two-chain lectins of Vicieae.
The two-chain and single-chain legume lectins exhibit high homology in the primary sequences and three-dimensional structures. Therefore evolution seems to have imposed only slight modification in the genetic coding for these lectins. This fact suggests the possibility of using lectins as an acceptable phylogenetic markers.
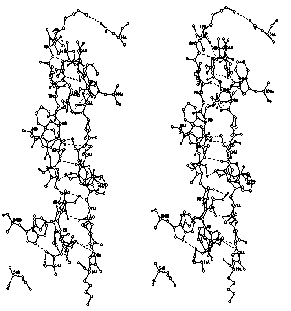
Stereodiagram of monomer-monomer interface in pea lectin.
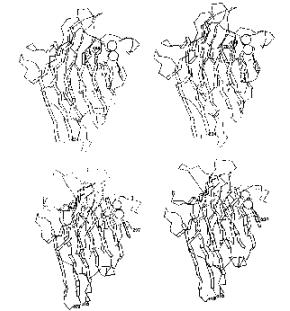
Stereodiagram of pea lectin monomer.
Lectins in Plant Tissues
Lectins in soybean plant are different from those in seeds. Dolichos bifluorus's lectins were studies in both the leaves and seeds. Lectins found to be produced with low level and constant for the period between 2-8 weeks after germination, then increased several folds in the next ten weeks. At this time extracts of stems and leaves contain several nanograms of lectin- like material per microgram of nitrogen, whereas mature seeds have about 1000 ng lectin per microgram. It is notable that in developing seeds the amount of lectin rises very abruptly. It is undetectable during the first 26 days after flouring but reaching a maximal level by day 28. Lectins from the stems and leaves don not agglutinate erythrocytes that could be agglutinated by seeds lectins. Stems and leaves lectin is a dimer of a molecular weight of 68,000, in contrast to the tetrameric seed lectin with a molecular weight of 110,000.
Toxicity of Lectins
Pathological lesions occur in animals injected with kidney beans extracts. Various tissues suffer from parenchymatous, fatty degeneration, and edema. In the liver local necrosis and fatty changes can be observed. Hemorrhages are observed in the stomach, the intestinal wall, and other organs. Distentions of capillary vessels may present in the Kidney and myocard with numerous thrombi. Morphological changes in rats fed navy beans include: increased weight of kidney and heart, pancreatic acinar atrophy, and fatty metamorphosis of the liver. Such changes may be attributed to the low availability of essential amino acids and low food intake of the animals consuming the raw bean diet. For example, rats fed raw kidney beans develop multiple histological lesions. Also, lectins from red kidney beans are found to induce small intestinal epithelial growth, crypt cell hyperplasia and DNA synthesis. Small amounts of isolated black bean agglutinin show low food absorption and nitrogen retention in rate.
The absorption of glucose from a ligated intestinal loop in anesthetized rats, previously fed a bean diet or given the black bean agglutinin by stomach tube, was much decreased. Raw kidney beans were found to interfere with vitamin E utilization in chicks. The hypoglycemia observed in rats fed a bean diet may indicate a reduced intestinal absorption of glucose.
Diet rich in raw soybean has a goitrogenic effect. This is indicated by the fact that fecal loss of thyroxine from the gut is higher in animals fed raw soybeans than in the controls. Raw soybean meals reduces fat and fatty acids absorption (not soybean trypsin inhibitor) in young chicks. Such meals also depresses the utilization of vitamin D in turkey. These effects are not found when the meals include heated soybean.
Ricin, abrin, crotin, and related toxins, produce similar macroscopic and microscopic pathological lesions. The intensive inflammation with destruction of epithelial cells, edema, hyperemia, and hemorrhages in the lymphatic tissues are very common. Several signs of toxicity may include: fatty degeneration and necrosis in the liver, degenerative lesions of the myocard, and extension and presence of blood clots of capillaries of all organs. At the site of lectin application, local hemorrhages are frequently observed.
In vitro, plant lectins effect lymphocyte mitogenesis, aggregate immunoglobulin induce histamine release from basophils and mast cells. When raw ground garden beans are supplemented with essential nutrients diet, weight lose and death to rats may occur within 1-2 weeks, due to the toxicity of the lectins in the beans. Raw navy beans have been found toxic for Japanese quail but not toxic for germ-feed birds. However, several cases of human intoxication were reported due to ingestion of raw or partially cooked beans.
Effect on the Gastrointestinal Tract
When given orally to experimental animals, lectins interact with the mucosa of the gastrointestinal tract causing acute gastrointestinal symptoms, failure to thrive and even death. When administered parenterally, they can alter host resistance to infection or to tumor challenge. They even can be highly allergenic under certain conditions. In vitro and in vivo tests show that intestinal cell damage are caused by bean lectins. In this regard, intestinal invertase is strongly inhibited by bean lectin as well as the absorption of vitamin B. One of the possible explanation of the toxicity of the lectins, which are resistance to gastric and intestinal digestion, is the binding to the cell lining of the intestinal walls, causing lesions and interference with nutrients absorption.
Effects on Cell Membrane
The reaction between the agglutinin and the cell membrane is believed to result in an alteration of the cell function thus producing the toxic effect. Only those cells bearing the specific receptor groups for the respective lectin would be effected. For instance, significant changes in membrane properties are induced by binding of lectins to liver cells of diabetic rats. The changes may influence such cellular properties as aggregation and deformability of erythrocytes, permeability, electrical resistance, and binding properties of mitogen, hormone, and lipoprotein receptors. The binding of concanavalin A and ricin, for instance, can be reduced by 20-25% as a result of a membrane reduction of glycoprotein carbohydrates content.
Ricin Toxicity
After intravenous injection of ricin, both the intestinal tissue and the intestinal juice of rabbits became highly toxic, indicating its concentrating in this tissue and its secretion into the intestinal lumen, but it can not be found in urine. Ricin may be appear in the milk of lactating guinea pigs which had been injected after the birth of the litters (suckling young became markedly resistance against subsequent injections of the toxin).
Magnesium blood level decreases after injection of ricin in cats. Quantitative analysis of plasma, liver, and urine, of rats acutely poisoned with ricin, along with the observation of the reduced respiratory quotient of the liver, can be used to conclude that: the toxic action of ricin may be explained by an interference with some metabolic process in the liver, possibly the Krebs cycle. Also, a rise of the blood values of urea, glucose, bilirubin, transaminases, and lactic dehydrogenase in rats fed ricin could be observed. However, detection of albumin and hematuria could lead to the conclusion that a hepatonephritis with hepatic cytolysis may be an early manifestation of ricin intoxication. A lag period between the injection of a lethal amount of ricin and death is not less than 12 hours. Ricin is many times more toxic when injected than when given orally.
Detoxification
The destruction of ricin toxicity by heat has long been recognized (1889 by Stillmark). The presence of more than one toxic principle which differ in heat resistance in castor beans must be taken into account when working with products derived from these seeds.
Although the castor bean allergen is more resistance to boiling than ricin, it can be inactivated by autoclaving. For its safe use as fertilizer and for animal feeding, detoxification of castor pomace is essential. Steam heating significantly reduces the toxicity of pomace to become harmless for sheep, rabbits, and rats, when the beans is about 10% of their diet. When used before heating castor bean, calcium hydroxide may aid in achieving complete destruction of the ricin and the allergen.
However, animals can be immunized successfully when injected with heated ricin solution in order to resist the toxicity of castor bean cake. Mice can also be protected against the fatal action of ricin if injected with blood serum from immunized goats (when given not later than 6 hours after the ricin injection).
Autoclaving is proved very helpful in enhancing the nutritive value of legumes, an effect that is probably related to the destruction of toxic hemagglutinins and other growth inhibiting factors. For complete elimination of the toxicity of kidney bean and field bean, preliminary soaking prior to autoclaving is required. In addition, autoclaving for 5 minutes is sufficient to eliminate the toxicity of finely ground navy bean meal.
However, dry heating has been found to be less effective. Thirty minutes of dry heating had little effect on hemagglutinating activity of certain varieties of V. vulgaris, and activity was still detectable after 18 hours of heating. Whereas, heating of the soaked beans or autoclaving was fully effective.
Moreover, formaldehyde has been recognized to reduce the agglutinating and toxic actions of ricin and agglutinating activity of bean lectin, but phenol was inactive in this respect. Potato lectin was more rapidly destroyed by phenol than by formaldehyde.
Adsorption of lectins on erythrocytes or stroma that are brought into solution is observed after heating to 56 degree C. The agglutinating and the toxic activity from a bean lectin solution disappear when it is treated with stroma. For both ricin and kidney bean agglutinin, no agglutination of erythrocytes occurs at low pH, and adsorbed lectins are observed to dissociate from the erythrocytes.
Lectins and Cancer
lectins present on the surface of tumor cells are targeted for therapeutic purposes. It has been found that treatment with anti- lectin antibodies can suppress growth of tumor cells in agarose, and inhibit lung colonization in vivo. Lectins have the potential use in cancer treatment strategies due to the fact that lectins present on the surface of tumor cells are capable of binding exogenous carbohydrate-containing molecules and internalize them by endocytosis.
For example, wheat germ lectin (WGA) is found to induce lectin- dependent macrophage-mediated cytotoxicity against human bladder cancer (T-24) cells. Alveolar macrophage (AM) are phagocytes, mainly present in the pulmonary alveoli, are important in the antitumor defense mechanism of the lung because they can bind to the target cell- but are unable to induce cytolysis. However, studies have revealed that human AM tumorcidal activity can be induce by wheat germ lectins. Another finding is that the sensitivities of six human tumor cell lines depend on the number of receptor sites exist on the surface of WGA. Although the effector mechanism is still unknown, the binding of AM with tumor cells initiated by WGA may increase sensitivity to the cytotoxicity mediated by human AM.
In addition, WGA is found to enhance the cell killing ability of murine peritoneal macrphages. In vivo studies show that WGA has an inhibitory effect on the growth of murine tumors. The tumoricidal activity of human blood monocytes can be induced by the WGA. As a result, the monocytes are able to become cytotoxic to four different human tumor cell lines: T-24 bladder carcinoma, A-375 melanoma, ACHN renal carcinoma, and U373MG glioblastoma. Murine systems also show similar response. However, concanavalin A, PHA, PWM and SBA are unable to produce tumoricidal monocytes.
References
Barondes, S. H. (1981). Lectins: Their multiple endogenous cellular
functions. Annual Review of Biochemistry, Vol 50: 207- 231.
Brownlee, M., and Cerami, A. (1981). The biochemistry of the complications
of diabetes mellitus. Annual Review of Biochemistry. Vol. 50: 385-432.
Cheeke, P., and Shull, Lee. (1985). Natural toxicants in feed and poisonous
plants. Avi, Inc. Westport.
Cooper-Driver, Gillian A. (1983). Chemical substances in plants toxic to
animals. In Miloslav Rechcigl, Handbook of Naturally Occurring Food Toxicants,
(pp. 213-240). CRC Press, Florida.
Etzler, Marilynn, (1983). Introduction . In Irwin Goldstein, and Marilynn
Etzler (Eds.), Chemical taxonomy, molecular biology, and function of plant
lectins, (pp. 1-5). Progress in Clinical and Biological Research, Vol.
138. Alan R. Liss, Inc., NY.
Jaffe, Werner G. (1969). Hemagglutinins. In Irvin Liener (Ed.), Toxic Constituents
of Plant Foodstuffs, (pp. 69-101). Academic Press, NY.
Jaffe, Werner (1983). Handbook of Naturally Occurring Food Toxicology.
In Miloslav Rechcigl, Handbook of Naturally Occurring Food Toxicants, (pp.
31-38). CRC Press, Inc., Florida.
Lotan, R. (1983). Differentiation-associated modulation of lactoside binding
lectins in cancer cells. In H. Gabius and S. Gabius (Eds.), Lectins and
Cancer, (pp.153-169). Springer-Verlag, Berlin.
Nachbar, M., and Oppenheim, J. (1980). Lectins in the United States diet:
a survey of lectins in commonly consumed foods and a review of the literature.
The American Journal of Clinical Nutrition, Vol. 33, No. 11, 2338-2345.
Ogawara, M., Utsugi, M., Yamazaki, M., and Sone S. (1985). Induction of
human monocyte-mediated tumor cell killing by a plant lectin, wheat germ
agglutinin. Japanese Journal of Cancer Research (Gann), Vol. 76, No. 11,
1107-1114.
Ogawara, M., Sone, S., and Ogura, T. (1987). Human alveolar macrophages:
Wheat germ agglutinin-dependent tumor cell killing. Japanese Journal of
Cancer Research (Gann), Vol. 78, No. 3, 288- 295.
Peumans, W., Nsimba-Lubaki, M., Broekaert, W., and Van Damme, E.(1986).
Are bark lectins of Elderberry (Sambucus nigra) and Black Locust (Robinia
pseudoacacia) storage proteins?. In Leland Shannon and Maarten Chrispeels
(Eds.), Molecular biology of seed storage proteins and lectins, (pp. 53-63).
The American Society of Plant Physiologists.
Pusztai, A. (1991). Plant lectins. Cambridge University Press, Cambridge.
Suddath, F., Parks, E., Suguna, K, Subramanian, E., and Einspahr, H. (1986).
The crystal structure of Pea lectin at 3.0 A resolution. In Leland Shannon
and Maarten Chrispeels (Eds.), Molecular biology of seed storage proteins
and lectins, (pp. 29- 43). The American Society of Plant Physiologists.